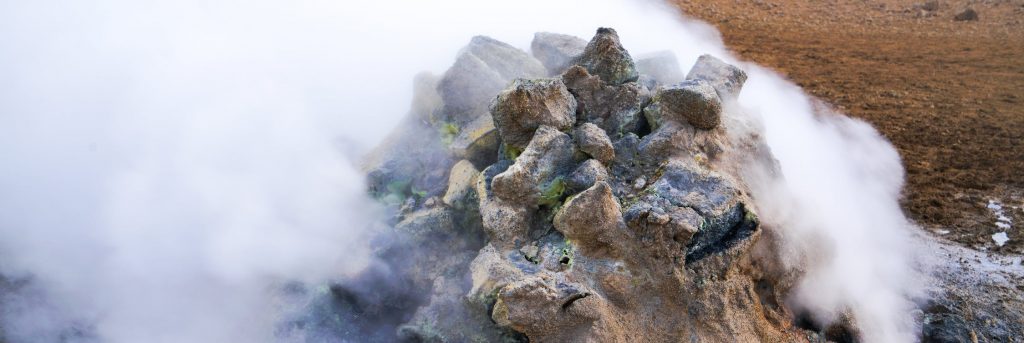
INTRODUCTION
Geothermal energy – by definition – is the energy stored in the form of heat in rocks and in vapours or liquids trapped in rock pores and fractures under the surface. The continuous renewal of geothermal energy is fed by the decay of radioactive isotopes found in the Earth’s crust. Geothermal energy has been a source of energy to humankind since the dawn of civilization.
Geothermal energy has many advantages:
- it is a renewable energy source, which is local
- it is widely available, since underground heat is global
- it is a base-load energy source, it provides 24/7 delivery with predictable outputs irrespective of weather conditions
- it has huge untapped potential which can boost economic growth
- it has numerous applications: in addition to power generation from very high temperature fluids and vapours, geothermal heating can supply energy at different temperatures from low (15-20 ℃) to high (100 ℃ or above). Furthermore multiple applications can be optimized by cascade-users of heat at progressively lower temperatures.
- its different loads (base load, or flexible adjusted to the actual demand) and capacities can be matched to different demands (from a few kWth to tens of MWth), thus providing extra flexibility for operators
- it can be combined with other energy sources to increase efficiency
- it has a low environmental footprint, as a resource it is invisible
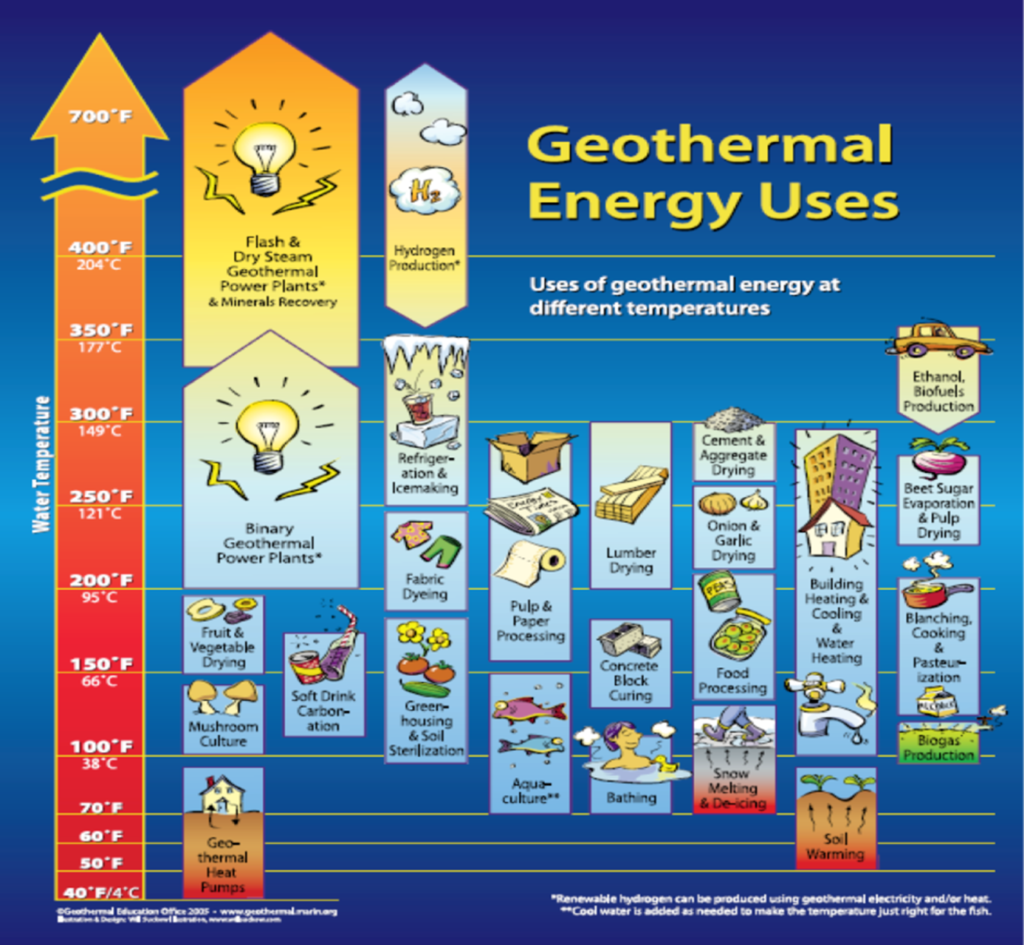
Fig. 1: Lindal diagram showing the multiple uses of geothermal energy at different temperatures
In line with the EU climate and energy policies, decarbonising heat consumption in homes, buildings and industry, as well as power production is the only way the European Green Deal can deliver emission reductions. The adoption of the EU Climate and Energy Package for 2030 plays a key role in paving the way to accelerate geothermal developments, as a sustainable alternative to fossil energy.
GEOTHERMAL POWER PRODUCTION
Geothermal power plants use steam coming from deep lying hot geothermal reservoirs. The steam rotates a turbine that activates a generator, which produces electricity. In flash steam power plants (Fig. 2) very hot water (around 180 ℃ or higher) flows upward through wells under its own pressure. During its ascent, the pressure of the fluid decreases and some of the hot water boils into steam. The steam is then separated from the water and used to power a turbine/generator. Any leftover water and condensed steam are injected back into the reservoir, making this a sustainable resource. These types of power plants – given by the required high temperature – are restricted to volcanic areas (e.g. Iceland, Turkey, Italy).
Binary cycle power plants (Fig. 2) operate on geothermal waters having somewhat lower temperatures of about 100-180°C with a working fluid compound with a low boiling point. The working fluid is vaporized in a heat exchanger and used to turn a turbine. The water is then injected back into the ground to be reheated.
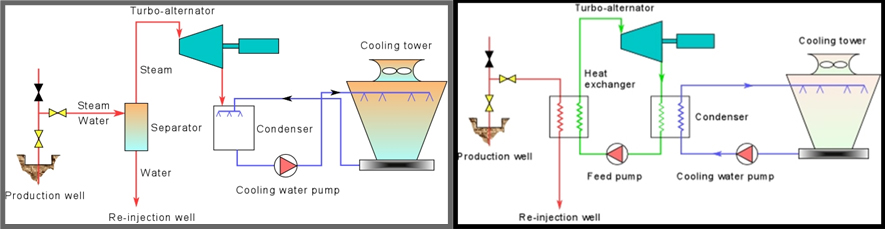
Fig. 2 Geothremal power production by flash steam (left) and binary (right) plants
The utilisation of geothermal electricity is expanding in Europe with a total of 3,3 GWe electricity generation capacity from 130 operating plants (2019), with the leading role of Turkey, Italy, and Iceland. The number of projects under development (36), or in planning (124) however indicate the interest to develop this clean, renewable and baseload energy source. From the EUSDR countries Croatia (17,5 MWe installed capacity) and Bavaria-Germany (altogether 7 plants with 4-6 MWe installed capacity each) have binary (ORC) geothermal power plants, while Austria, Hungary and Romania have some small scale (0,5-3 MWe) pilot plants in operation.
GEOTHERMAL HEATING
There are two ways of extracting geothermal energy from the ground for direct uses (i.e. heating purposes)
- by heat recovery (closed loops down to a depth of a tens to hundreds of meters, i.e. deep geoprobes – not discussed at further details)
- by extracting thermal water
The extraction of thermal water can happen in two different ways:
- During direct thermal water utilization, the extracted thermal water itself is circulated in the heating systems. This can happen if the thermal water has favourable chemical properties, e.g. it has a low content of dissolved material and thus does not cause scaling, it is not corrosive, or it has a low free gas content.
- During indirect thermal water utilization, the primary geothermal loop transfers the heat via heat exchangers to a secondary heating loop circulating “clean” water; it is the latter which provides the heat for consumers. This solution is preferable when the extracted thermal fluid cannot be fed directly into the heating system due its unfavourable chemical characteristics.
After utilization, the used and cooled thermal fluid can be discharged on the surface (into a sewage or rainwater system, to an open surface channel, to streams, or rivers), or can be returned back into the aquifer (reinjected). Surface discharge has some possible severe consequences, e.g. the salinisation and increased thermal load of natural surface waters. Moreover the lack of reinjection can cause the depletion of the geothermal aquifers, reflected in a significant reduction of the water level and/ or a fall in pressure in the production wells. However, by using reinjection these problems can be eliminated. Consequently, production becomes renewable and sustainable from the water-balance point of view (the carrying medium of the heat); furthermore, it also becomes sustainable with respect to energy, since the reservoir is replenished and the reinjected cool water warms up again in the subsurface if the flow rates are not too large.
Typical cases of geothermal energy usage for heating purposes are the following:
- Geothermal district heating (geoDH): this is frequently found in big cities with an existing district heating network and heating stations, originally fed by fossil fuels. In some cases these systems (or parts of them with certain heat loops) are supplied by thermal water (Fig. 3). In thermal water town heating systems a few buildings (typically public buildings, such as the town hall, hospitals, schools, libraries, etc.) are heated by thermal water through a specially designed thermal water pipeline loop; the latter connects the production
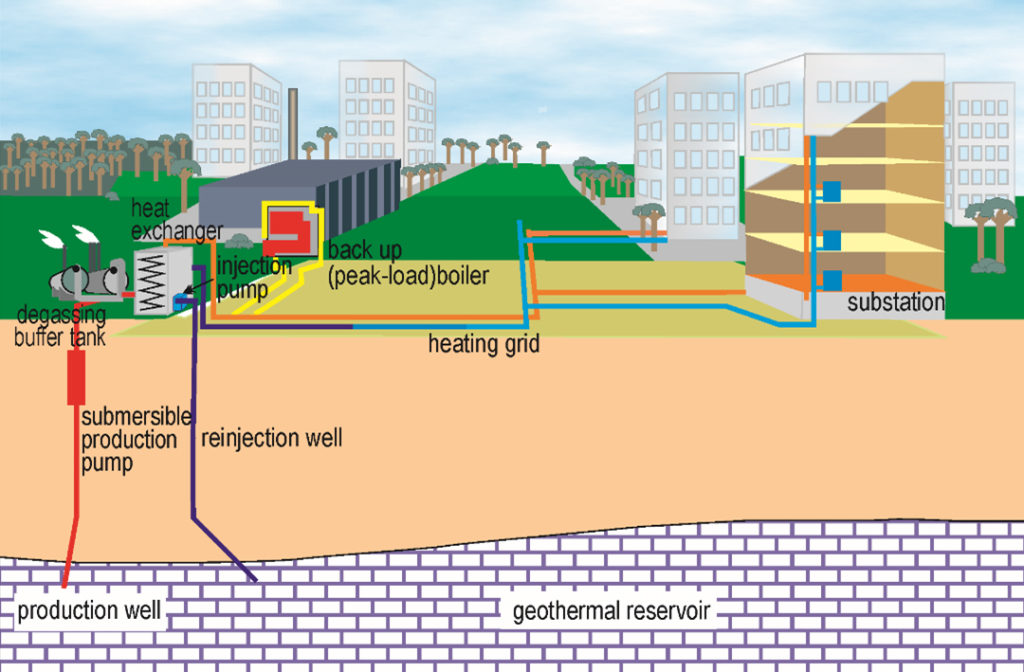
Fig. 3 Geothermal district heating
- Individual space heating: this is common in spa complexes where the heat content of the produced thermal water for balneology is used to heat the associated buildings (most commonly through heat exchangers). Often, some of the water is also used for sanitary water heating, or as the sanitary water itself.
- Agricultural use: in this particular area thermal water can be used for a great variety of purposes: heating of greenhouses, plastic tents/polythene tunnels, stables, hatcheries, soil heating, fish farming etc. (Fig. 4).
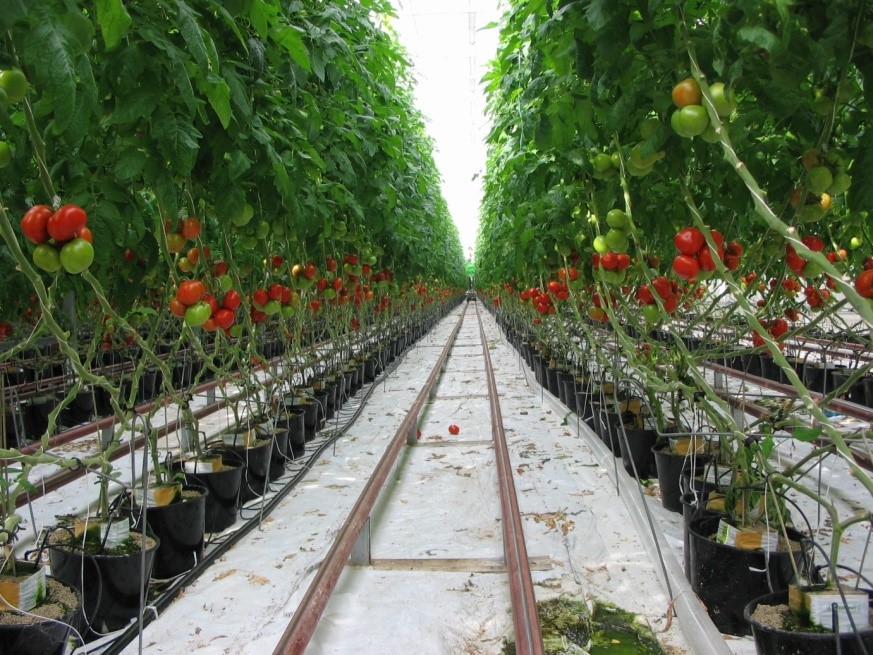
Fig. 4: Geothermal heating of a greenhouse
In order to maximize the efficient utilization of the heat content of the extracted thermal water, cascade systems may be applied. In such systems heating circuits with various temperature differences are aligned in order to maximize the specific heat capacity of the thermal water. The secondary medium of one district appears as the primary medium in another district, in line with the lowering of temperature demand (Fig. 5).
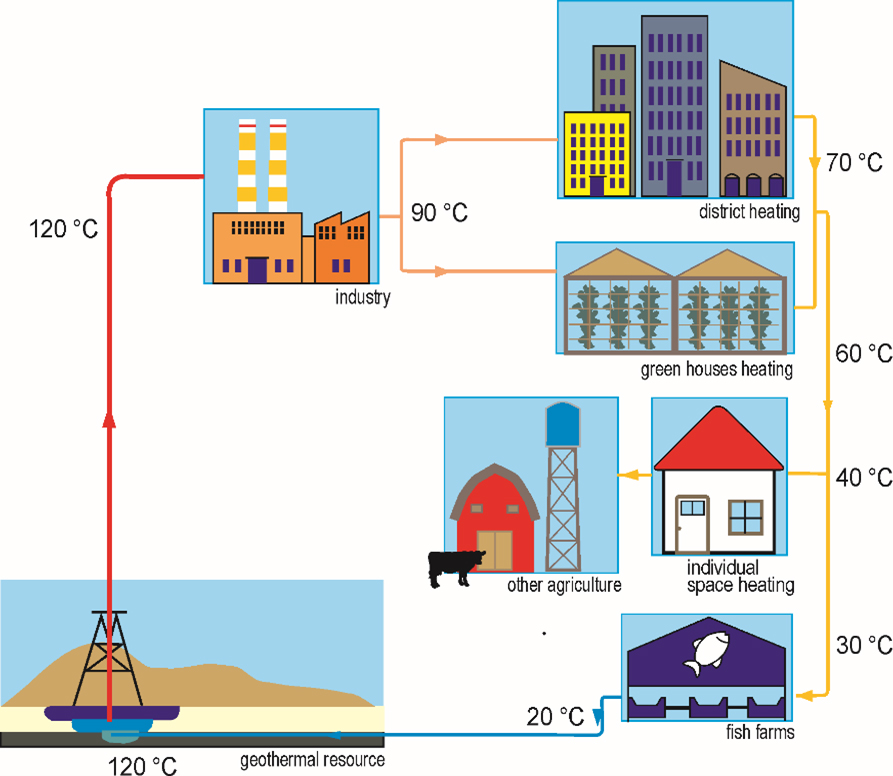
Fig. 5: Cascade use of geothermal energy
Geothermal district heating and heat uses in industry, agriculture and other sectors represent a 5,5 GWth installed capacity in 327 systems in 25 European countries (2019). The leading countries are France, Germany and Iceland, However Hungary with its 908 MWth installed capacity and 2267 GWth/y annual production (2018) has been keeping its 4th place on the European list for some years. Some other EUSDR countries (Romania, Slovakia, Serbia) are among the top 10, and most countries have significant untapped potentials (e.g. Slovenia, Croatia, Austria).
POLICY BACKGROUND
- EU Strategy for Heating and Cooling (2016) COM(2016) 51 final
- EU Directive 2012/27/EU on energy efficiency
- EU Directive 2010/31/EU on energy performance of buildings
- EU Ecodesign and Energy labelling framework
- Directive 2009/28/EC on the promotion of the use of energy from renewable sources
- Renewable Energy Directive (RED II)
- Water Framework Directive (2000/60/EC)
- Groundwater Directive 2006/118/EC
- Directive 2011/92/EU on Environmental Impact Assessment- EIA
- EU climate targets for 2030
- Paris Agreement (2015)
- Energy Union Strategy (2015)
- “Clean Energy for All Europeans” package (2016)
- European Green Deal
- Recast of the Renewable Energy Directive (RED II) (2018)
- European Climate Law COM(2020) 80 final
- A Renovation Wave for Europe – greening our buildings, creating jobs, improving lives (2020): COM(2020) 662 final
- Powering a climate-neutral economy: An EU Strategy for Energy System Integration (2020): COM(2020) 299 final
- EU Emissions Trading System (EU ETS)
- EU Effort Sharing Mechanism
LIBRARY
- EGEC Geothermal Market report 2018
- Cascades and Calories: Geothermal Energy in the Pannonian basin for the 21st Centry and Beyond (DARLINGe project book) (2019)
- Strategic Research and Innovation Agenda, European Technology and Innovation Platform on Deep Geothermal (2019)
- Vision for Deep Geothermal, European Technology and Innovation Platform on Deep Geothermal (2020)
- Report on Competitiveness of the geothermal industry, European Technology and Innovation Platform on Deep Geothermal (2019)
- Geothermal energy: the bedrock of energy transition (EGEC brochure) (2021)
- Strategic Research Innovation Agenda for Geothermal Technologies, European Technology and Innovation Platform, Renewable heating and Cooling (2020)
- Implementation Roadmap for Deep Geothermal, European Technology and Innovation Platform on Deep Geothermal (2019)
- Renewable Energy Policies in a Time of Transition, Heating and Cooling, IRENA (2020)
USEFUL LINKS
- EGEC – The Voice of Geothermal in Europe
- International Geothermal Association
- European Technology and Innovation Platform on Deep Geothermal
- International Renewable Energy Agency
- European Technology and Innovation Platform on Renewable Heating and Cooling
- Geo-ERA-Geonergy
- ThinkGeonergy
- European Energy Research Alliance – Geothermal
- European Commission/ Energy/ Renewable Energy
- Danube Region Geothremal Information Platform (DRGIP)