INTRODUCTION: Drivers for hydrogen use
To become climate-neutral by 2050 – in line with the European Green Deal and with the EU’s commitment to global climate action under the Paris Agreement – Europe needs to transform its energy system, which accounts for 75% of the EU’s greenhouse gas emissions. Hydrogen – as an energy vector – can support the decarbonisation of industry, transport, power generation and buildings, and can power sectors that are not suitable for electrification, furthermore it can play a systemic role in the transition to renewable energy sources by providing a mechanism to flexibly transfer energy across sectors, time, and place.
THE HYDROGEN VALUE CHAIN
The hydrogen value chain – like all energy systems – can be divided into three main parts: production, storage and transport, and end use. It is important to notice, that roundtrip efficiency must be considered at all parts, as every transformation step in the hydrogen supply chain has associated energy losses and reduces the efficiency of the overall chain.
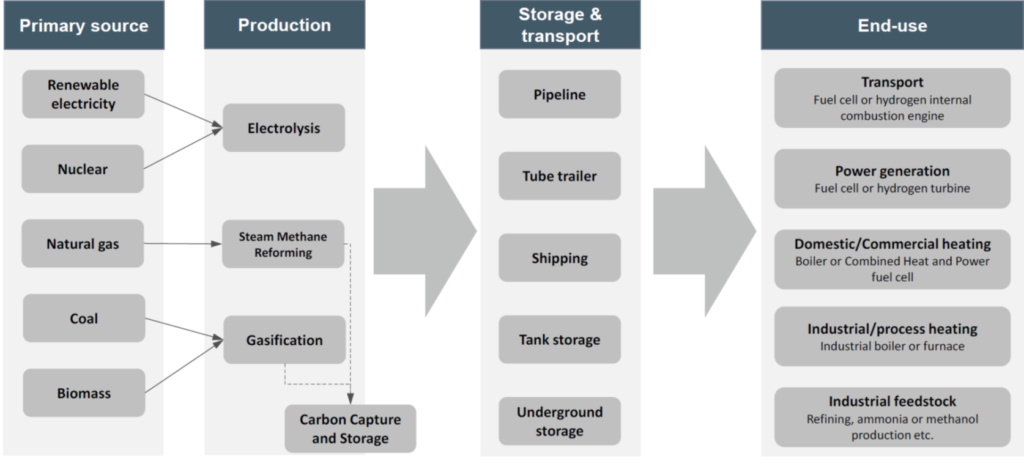
Production
Hydrogen is typically found in combined chemical forms such as water (H2O) or in methane (CH4). Consequently, a hydrogen-based energy cycle must first start with the production of hydrogen which requires the input of large amounts of energy to separate hydrogen from these molecules.
In the electrolysis process, water is split into its component elements using electricity and the hydrogen is subsequently separated from the oxygen. Several types of electrolyser exist and are categorised by the type of electrolyte they use. The electrolysis of water using renewable electricity produces ‘renewable hydrogen’ which is referred to as “green hydrogen”. The optimal siting of production facilities (i.e. proximity of high photovoltaic and wind capabilities) may lower the costs of the hydrogen value chain and counterbalance the relatively high costs of hydrogen storage and transportation.
Hydrogen can be also produced by biomass gasification – this is also considered as renewable hydrogen. There are two main routes; dry biomass can be gasified by being heated and combined with oxygen to produce syngas (a mix of mostly hydrogen and carbon monoxide), or syngas can be produced from anaerobic digestion of wet biomass. The processes are carbon neutral since the CO2 released was previously absorbed by the biomass as it grew. Biomass gasification is more costly than SMR (see below) and relies on a consistent supply of biomass, therefore raises sustainability concerns over the of the use of arable land.
Hydrogen is currently produced predominantly from fossil fuel sources by steam methane reforming of natural gas (SMR), or gasification of coal. These processes results in CO2 emissions, therefore it is called as “grey hydrogen”. Carbon capture and storage (CCS) may be added to traditional SMR resulting in capture of 90% of the CO2, thus making it also a low carbon hydrogen, frequently referred to as “blue hydrogen”. Although CCS adds cost to the hydrogen produced, it is recognised that this low carbon hydrogen will likely play a key short term role in developing a hydrogen economy until zero carbon, renewable hydrogen becomes cost competitive, in spite of the fact that the cost of natural gas is likely to increase as carbon pricing becomes more prevalent.
A wide range of other less mature technologies for hydrogen production also exist.
The cost of hydrogen production is expected to lower by 2030 and beyond, as hydrogen systems are scaled up and technologies, manufacturing and operations mature, however largely depend on production methods, their efficiency, location, CCS availability, etc.
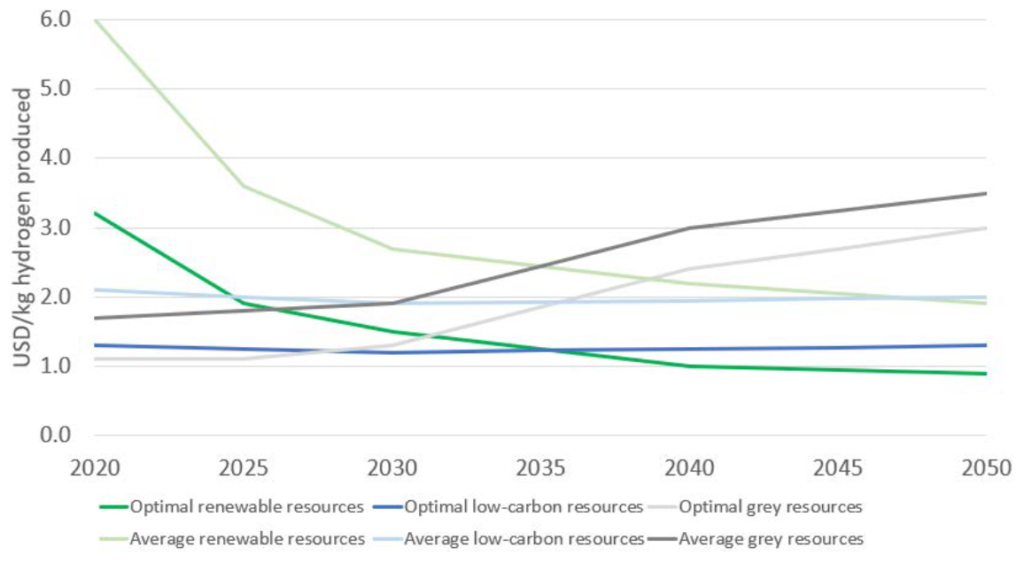
Transport and storage
Hydrogen must first be conditioned for transportation into either compressed gas, a liquid, or combined with other elements in a hydrogen carrier. In one of these forms it can then be carried in multiple ways, e.g. in pipelines, which can be existing (retrofitted) gas grids where blended hydrogen with natural gas can be transported without the need for system modifications up to 20% v-v (volume-volume). It is expected that natural gas transmission and distribution pipelines will be the lowest cost option in moving significant amounts of hydrogen from production source to end user. Nevertheless there are already plans for dedicated hydrogen pipelines in Europe, however the capital costs of building new transmission pipelines is a significant investment. Gaseous or liquid hydrogen can be also transported in smaller amounts in specially conditioned (pressure and temperature) trucks or wagons, or in larger volumes by waterborne vessels, either on inland waterways or the high seas, similar to liquefied gas (LNG). These transportation methods might be employed in regions where no pipelines exist, as it offers much more flexibility and lower capital expenditure.
Transportation has several technical challenges, such as reaction of hydrogen with metals, which affect pipeline and trucking materials and compressor designs, or potential leakage due to the very small hydrogen molecule size.
Hydrogen can be stored in much the same ways that it can be transported (compressed gas, cryogenic liquid or carrier molecules) with the chosen method dependent on factors such as the space constraints, the length of storage time required, etc. also considering that the volumetric energy density of hydrogen is low relative to fossil fuels. The cost of storing compressed hydrogen at low pressure in tanks is expected to be more expensive than large-scale underground storage, but not all regions are geologically suitable to accommodate underground storage (e.g. salt caverns, or properly sealed porous rock formations).
The insufficiencies in the current methods/infrastructure for efficient transportation and storage of large quantities of high density hydrogen are hindering the widespread implementation of the potential “hydrogen economy”.
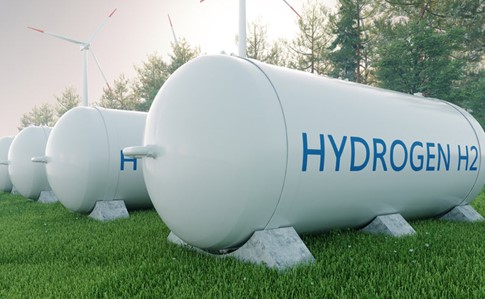
End-use
Industry
Most hydrogen today is used as a feedstock for industrial processes such as ammonia synthesis, oil refining and food manufacture. As a feedstock, it is the molecular properties of hydrogen that are required rather than its energy carrying ability. Direct substitution with zero or low carbon hydrogen in these processes are likely to be the first use cases for clean hydrogen.
Hydrogen can be used in other industrial processes, such as methanol synthesis, or steel production, however the latter requires major modifications to the whole plant, so it is likely to be a preferred application once the hydrogen economy has matured.
Hydrogen could also be used as a source of heat in industrial processes where a high flame temperature is beneficial (e.g. cement production).
Transport
Fuel cell electric vehicles (FCEV) use hydrogen as a fuel and convert it to electrical energy in a fuel cell. FCEVs compete with battery electric vehicles (BEVs) with the main advantages being range (500 km+) and refuelling time which is roughly comparable with gasoline or diesel refuelling. These properties make FCEVs highly advantageous for buses and trucks. The current constraint is the availability of hydrogen refuelling stations. Further challenges include the limit on global capacity to produce the lithium and cobalt required by these batteries, as well safety concerns.
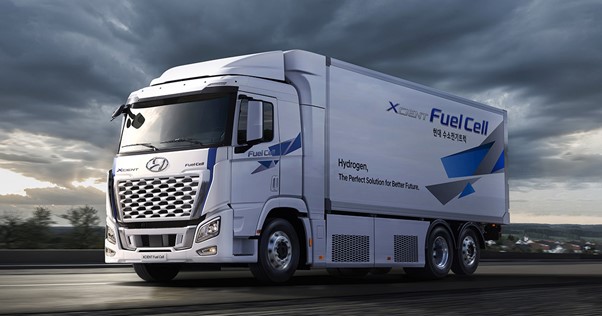
The use of hydrogen in trains is also being explored in some countries (Germany, UK, Japan). Where train routes have already been electrified, hydrogen is unlikely to be competitive, nevertheless conversion of diesel trains to hydrogen-powered trains may be less expensive and feasible than electrification.
The use of hydrogen to power ships is also under consideration, especially for short ferry routes. For longer deep sea routes, liquid hydrogen may be an alternative, although hydrogen-based fuels, such as ammonia may be better suited to these applications.
Hydrogen is also being considered as a possible aircraft fuel in conjunction with fuel cells, particularly if the challenges of how to store high density hydrogen onboard can be overcome.
Heating
Hydrogen can be used for space and water heating either blended with (up to a 20% volume-volume basis no modification of the gas appliances is required), or as a substitute for natural gas. However, the greenhouse gas emissions benefit is limited given the low volumetric energy density of hydrogen. Hydrogen heating can be cost-effective relative to electricity-based solutions, especially where a highly developed gas network already exists. Furthermore hydrogen networks are able to store energy within the network and respond rapidly and effectively to changes in demand. It is expected that cheaper low carbon hydrogen from natural gas would be used in the first instance for heating, while renewable hydrogen will likely be reserved for higher value applications, like transport.
Power generation
Hydrogen / blends can be also used as a gas-turbine fuel, but not all gas turbines are suitable for retrofit modifications. Constrains are due to hydrogen’s high flammability and flashback, as well as its higher NOx emissions. However hydrogen can be used to generate grid power and could prove to be a viable solution for providing consistent power to the electricity system, allowing more effective integration of intermittent renewables. As with heating, it is generally considered that in the short-term, low carbon hydrogen would be used in power generation over renewable hydrogen as it is generally lower cost.
Storage and sector coupling
One further potential application for hydrogen is in energy storage and system balancing. Much of the renewable electricity generation potential is intermittent and non-dispatchable and requires flexibility in the system in order to incorporate it. System flexibility can be delivered through storage, demand side response and greater interconnectivity, which can ensure that differences in load and generation in different regions balance out. Converting excess electricity into hydrogen and its inter-seasonal storage (Chapter 2.2) can complement fluctuating energy demands between summer and winter.
E-fuels
Another application for hydrogen is in the production of electrofuels (e-fuels), which are produced using electricity from renewable energy sources to make renewable hydrogen, which is then combined with CO2, but also biogenic sources, to produce synthetic hydrocarbons. Despite some disadvantages (e.g. high costs, low efficiency, sustainability concerns of biogenic sources) for some very hard-to-abate sectors like long distance marine or air transport, e-fuels do offer a promising alternative.
HYDROGEN POLICY FRAMEWORK
While the primary objective of policies to support hydrogen is decarbonisation, other important drivers include the need to improve air quality especially related the road transport as well as economic development through the establishment of hydrogen-related activities. That’s why hydrogen as part of industrial strategy features heavily in the hydrogen roadmaps.
The European Commission has identified that hydrogen will be a key instrument for meeting the Green Deal objectives to achieve a ‘climate neutral’ Europe. An important pillar is smart sector integration, i.e. bringing closer the electricity, gas and heating sectors ‘in one system’; and hydrogen is at the heart of this system transformation.
The EU Hydrogen Strategy is laying out the ambition to decarbonise hydrogen production and to expand its use in sectors where it can replace fossil fuels. The Strategy addresses how to transform the hydrogen potential into reality through investments, regulation, market creation and research and innovation. The priority is to develop renewable hydrogen, produced using mainly wind and solar energy. Two key production targets are the roll-out of 6 GW of electrolyser capacity and 1 Mt of production by 2024, increasing to 40 GW of installed electrolyser capacity and 10 Mt of production by 2030. In the short and medium term other forms of low-carbon hydrogen are needed to rapidly reduce emissions and support the development of a viable market. From 2030 to 2050, renewable hydrogen technologies should reach maturity and be deployed at large scale across all hard-to-decarbonise sectors.
Nevertheless to reach these ambitions a review of a substantial number of legal acts (e.g. RED II, the Energy Efficiency Directive, TEN-E) is required. Furthermore the EU Hydrogen Strategy is expected to be translated into new legislative proposals including a common low carbon threshold/standard for the promotion of hydrogen production installations, comprehensive terminology and European-wide criteria for the certification of renewable and low carbon hydrogen (i.e. Guarantees of Origin), and a review of the legislative framework to design a competitive decarbonised gas market to be fit for renewable gases (i.e. the gas acquis reform).
EU INITIATIVES
The EU research and development programs focusing on hydrogen in the past Horizon 2020 framework program were supported by the Public Private Partnership named Fuel Cells and Hydrogen Joint Undertaking (FCH JU). The FCH JU is made up of three partners that is the European Commission, the Hydrogen Europe association and the Hydrogen Europe Research association. Hydrogen Europe brings together diverse industry and research players, large companies and SMEs, who support the delivery of hydrogen and fuel cells technologies. Hydrogen Europe Research is an association of universities and Research & Technology Organisations that are active in the hydrogen and fuel cell fields. One of its key documents is the Hydrogen Roadmap Europe, which is the first comprehensive quantified European perspective for deployment of hydrogen and fuel cells in two scenarios and depicts a full picture on how the use of hydrogen at a large scale can help the EU’s decarbonisation objectives.
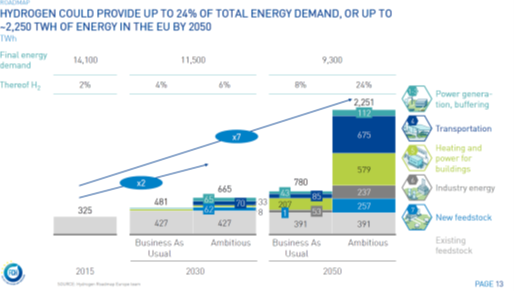
In the new framework program, Horizon Europe, the Clean Hydrogen for Europe Partnership replaces the FCH JU. The new partnership aims to accelerate development and deployment of European clean hydrogen technologies, contributing to a sustainable, decarbonised and fully integrated energy system and strengthen EU’s global leadership role through a € 2.0 bn research program, which is detailed in its Strategic Research and Innovation Agenda. The SRIA is structured along three pillars and seven specific objectives, which are broken down in clearly defined, concrete, operational roadmaps.
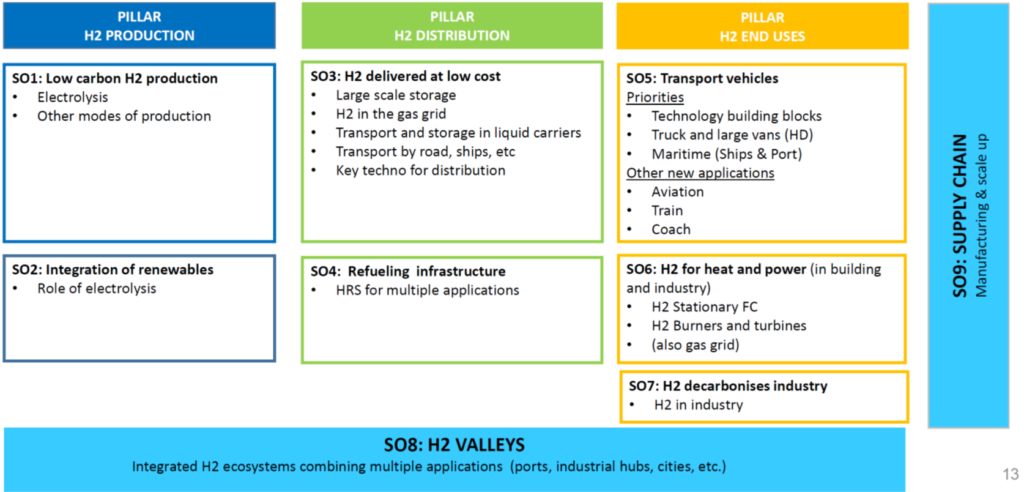
To help deliver on the European Hydrogen Strategy, the Commission has established the European Clean Hydrogen Alliance (ECH2A)with industry leaders, civil society, national and regional ministers and the European Investment Bank. The Alliance will build up an investment pipeline for scaled-up production and will support demand for clean hydrogen in the EU.
A special framework for future large-scale, cross-border hydrogen projects is the Important Project of Common European Interest (IPCEI) that enables state aid funding for large cross-border projects. The European Hydrogen Strategy foresees the uses of the IPCEI framework for hydrogen-related projects. One of these projects (Green Hydrogen@Blue Danube) has special relevance to EUSDR, as 2 GW wind and solar-based hydrogen production, transportation by barges on River Danube, and supply to industry and mobility hubs is envisaged in the Danube region.
LIBRARY
https://www.iea.org/reports/the-future-of-hydrogen
https://www.irena.org/publications/2019/Sep/Hydrogen-A-renewable-energy-perspective
https://www.clean-hydrogen.europa.eu/media/publications_en
https://hydrogeneurope.eu/industry/tech-descriptions/