INTRODUCTION: The need for energy storage
For many years, energy storage was not considered as a priority in energy systems, partly because the technologies were not yet economically viable, partly because the benefits of storage were valued less in centralized fossil fuel-based energy systems. Nevertheless, this situation is rapidly changing due to the cost-performance improvements in energy storage technologies and the global and EU political commitment to the transition of a low-carbon economy with a significant increase of share of renewables in the electricity generation.
Various energy scenarios all predict the significant restructuring of the global energy systems in the coming decades (Fig.1): the energy mix of primary energy consumption is expected to be rearranged and power consumption will significantly intensify by the end of 2030s. In the transition towards a lower-carbon energy system, renewables and natural gas will play major roles with the fastest growth of renewables: its share in primary energy consumption will increase from 4 % today to around 15 % by 2040.
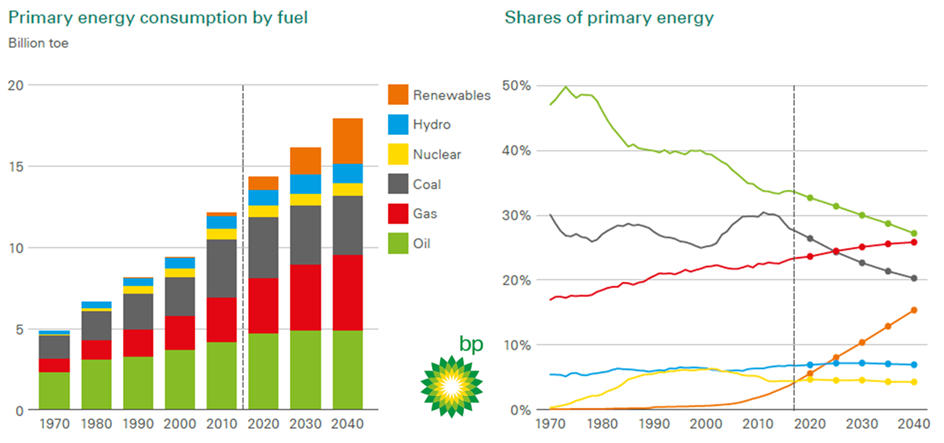
Fig. 1: Primary energy consumption by fuel and shares of primary energy (source: BP Energy Outlook 2019)
However, energy supply from renewable sources like wind, or solar power is subject to strong natural temporal fluctuations and therefore frequently does not match the instantaneous energy demand and energy base load (Fig. 2). Energy storage is thus required to dampen these fluctuations and to compensate for times of low power production. Energy storage will enable to optimize the integration of renewable energies into the electricity and heat systems. It will also facilitate grid interconnectivity and flexibility, and development of demand response functionalities. Furthermore it can also contribute to the decarbonisation of the transport sector by producing green fuel and can produce green raw materials for the chemical industry, like hydrogen. Energy storage methods therefore offer sustainable, predictable and long-term solutions to achieve the EU 2030 energy targets in line with the goals of the Paris Agreement.
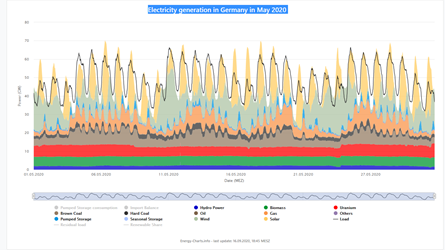
Fig. 2: Intermittent renewable electricity production highlighting daily fluctuations of solar and wind energy (source: Fraunhofer, https://energy-charts.info/
ENERGY STORAGE TECHNOLOGIES AND APPLICATIONS
Due to the fluctuating nature of renewable power production, storage is required on a wide range of time scales, ranging from seconds to months, as well as storage sizes, ranging from tens of kWh to tens of GWh (Fig. 3).
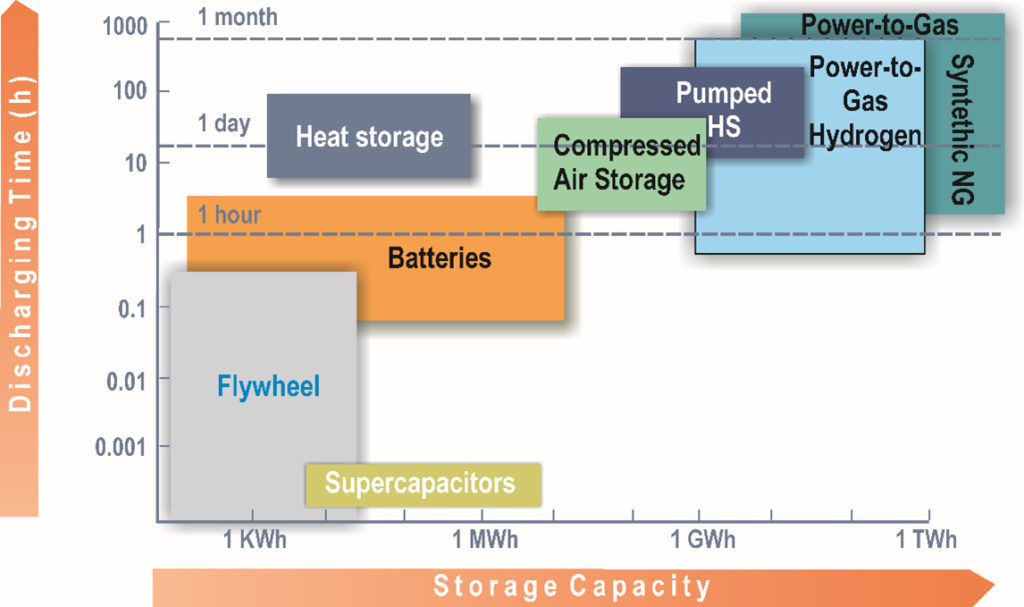
Five main available groups of energy storage technologies can be distinguished (EASE 2016) which varies according to their discharging time and storage capacity.
Mechanical energy storage
Compressed air energy storage (CAES)
CAES is a technology where excess electricity is used to drive compressors in adiabatic, diabatic, or isotherm way to pressure air. The compressed/pressurised air can be stored in underground caverns, or other (above surfaces) tank(s). During discharging, the air from the cavern or tank(s) is released and drives a turbo, or piston expander.
CAES plants can be used to provide grid scale storage services on a daily, or even weekly basis. There are only 2 such plants existing worldwide (USA and Germany), which makes these small-scale applications expensive. Moreover, these plants use natural gas for preheating of the compressed air before expansion, thus producing some amounts of CO2. Furthermore CAES has a relatively low round-trip efficiency (40 to 54%).
Kinetic energy storage (Flywheel)
This is a fast energy storage technology, where electricity is stored as kinetic mass in a rotating flywheel. It can be installed at any location, and is characterized by high power and energy densities. Flywheel is a mature technology completely introduced in the industrial market. Nevertheless, it is a short-term solution to store energy with low storage capacity and short discharging time.
Hydropower (pumped hydro storage)
This proven technology – which uses the potential energy of water – is the most efficient and flexible largescale means of storing energy available today. There is about 1000 GW of hydropower installed worldwide with an average annual production of about 3500 TWh, where 480 TWh are produced in Europe from an installed capacity of 160 GW. In pumped hydro storage, water is pumped from a low level reservoir via turbines to an uphill reservoir with the help of surplus electricity during off-peak periods (Fig. 4). When power consumption increases, water flow direction is reversed, and in turn, the uphill reservoir water drives the turbines to regain electricity. The estimated efficiency ranges from 70 % to 85 %. Capacity is between 1-10 GWh per cycle and is a massive storage method in both the short and medium term.
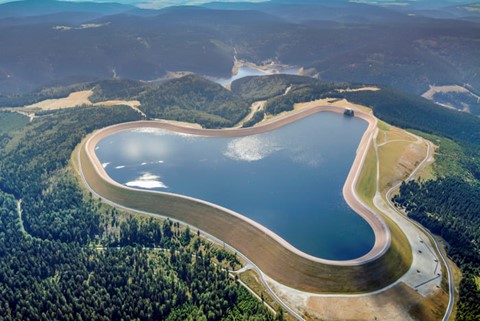
Fig. 4: An aerial view of the Goldisthal Pumped Storage Station, Germany, one of the largest hydroelectric power plants in Europe
Electorchemical energy storage
(also known as Battery-storage)
Batteries are based on single electrochemical cells that use chemical reaction(s) to create a flow of electrons – electric current. Each cell has voltages in the range from below 1 V up to about 4 V that can be combined in series to yield very high voltages if required (Fig. 5). Today the most commonly used technologies on the market available are Lead-based, Lithium-based, Nickel-based and Sodium-based batteries, which are used and tailored for a variety of different applications, most commonly as uninterruptible power supplies in grid balancing and mobile applications (e-cars). Batteries are also important strategic components in several key functions of the modern society, such as communication, mobility and defence.
Although batteries are still not very efficient as to their price/capacity ratio, and they also raise environmental degradation concerns (mining processes, waste prevention and recycling), as well as supply of critical raw materials (lithium), they are viable enough to be used on a large scale. Conventional (lead-acid and alkaline) batteries may provide even up to 40 MWh for load levelling. Advantages of batteries include flexibility and efficiency (in some cases near 100%); while its disadvantages are limited stored energy and power performance, lifetime, charging capabilities and costs.
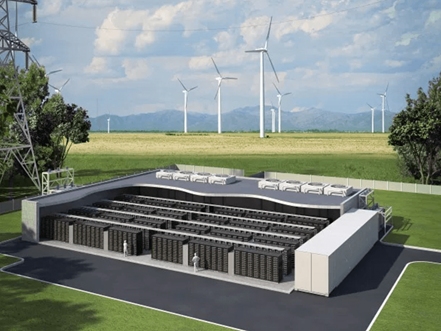
Fig. 5: Batteries in grid-scale energy storage
Chemical energy storage
This well-established technology uses renewable electricity to create chemical materials, mostly gases (Power-to-Gas) (Fig. 6). A hydrogen-based chemical storage system is a three-step process of (1) converting surplus renewable electricity to hydrogen using electrolysis; (2) storing the chemical energy as hydrogen, or synthetic methane in either the natural gas pipeline, local storage tanks, or even subsurface in salt caverns, or well-sealed porous formations; and (3) discharging the stored energy for mobility (hydrogen fuelled electric vehicles), or power through a gas turbine generator, or for various industrial applications. The main advantages of Power-to-Gas are the rapid, dynamic response of the electrolyser to integrate intermittent renewable sources of generation, and the unparalleled massive storage capacity of the existing natural gas infrastructure. Surplus electricity can be stored on short- (days) to long- (weeks/months) term without the need to discharge it, providing a seasonal storage capacity and the flexibility to discharge the stored energy. It also provides an opportunity for sector-coupling (a bridging technology between electricity and natural gas markets).
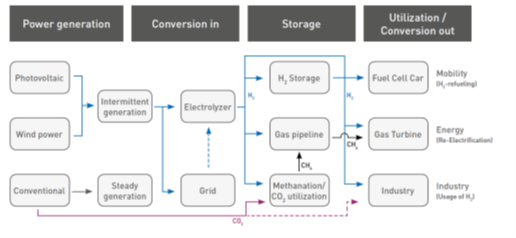
Fig. 6: Schematic overview of chemical storage pathways. Source: EASE / EERA Energy Storage Technology Development Roadmap 2030
The efficiency of the complete conversion chain from renewable energy via electrolysis to
hydrogen/methane, or other fuels and back to electricity can amount up to 40%, a level that
is also typical for conventional coal fired steam power plants.
Chemical storage is not a mature technology yet; the major challenges for its uptake include the (1) high CAPEX especially of the electrolysers, (2) system integration of the electrolyser, (3) safe and compact storage of hydrogen and the admixing limit in the natural gas grid, (4) improvement in efficiency.
The Power-to-Gas applications are best suited to large- very large scale systems (10-100 MW capacity) with seasonal storage capability. These large-scale and centralized energy storage solutions can provide flexible bulk power management services for electricity, gas and heat commodities.
Hydrogen Energy Storage has a potential in depleted gas fields with adequate sealing rock capacity. Some pilot projects have already been launched to test underground salt caverns for hydrogen storage as well.
Thermal energy storage
Thermal energy storage (TES) is a key element for effective generation and utilisation of heat, where heat supply and heat demand do not match in time, space, temperature and power. Heat sources may come from waste combustion, waste water, combined heat and power, as well as from other sources of surplus heat (geothermal, industry, datacentres, etc.). There is a wide range of diverse applications that require different temperatures, energy/power levels and use of different heat transfer fluids. Each storage concept has its best suited materials and these may occur in different physical phases: liquids (mostly water), molten salts, and solids are used as heat storage media. The thermal conductivity of the materials is important for the charge and discharge power of the storage system.
By use of underground thermal energy storage (UTES) systems, excess heat during summer is stored in the ground, to be extracted during winter (seasonal storage). One typical and relatively widespread application is a combination of a ground-source heat pump with aquifers (Fig. 7) for space heating and for sanitary hot water generation in the winter and for cooling in summer. High-temperature ATES systems use aquifers, as storage media, where storage temperature range between 50 and 150 ℃ and the storage depth between <100 to >500 m. Storage capacities are in the range of a few thousand MWh up to 20 000 MWh.
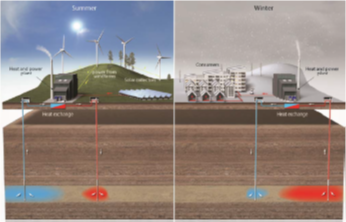
Fig. 7: The concept of aquifer thermal energy storage (ATES)
Thermal energy can be also stored in the subsurface in specially designed single-, or double U-tubes or coaxial pipes placed in boreholes (Fig. 8), these closed-loop systems use the soil volume as storage media. Storage temperature range between 45 and 80 ℃, and the storage depth between 30 and 150 m. Storage capacities range from 100 to 3800 MWh. Storage efficiency is between 45 and 60%.
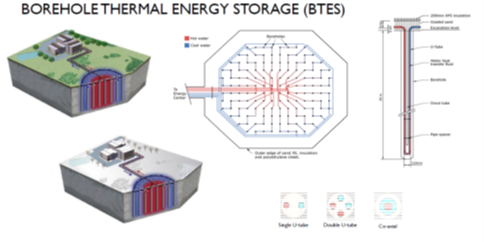
Fig. 8: The concept of borehole thermal energy storage (BTES)
The third way of storing thermal energy in the subsurface is the utilisation of mine water of abandoned and flooded mines, as low-temperature energy source for heating buildings. This method is used so far in Germany and the Netherlands.
A special case is where thermal energy is stored in water, displaced on the surface in large, properly insulated pits (pit thermal energy storage – PTES), as in some cases in Denmark. Storage temperatures are up to 90 ℃ and storage capacities range from 3000 to 12000 MWh. Storage efficiency may be up to 90% (both on short- and long-term), and these systems have high charge/discharge capacities.
In addition to water (liquids), natural materials in the form of rocks and pebbles are suitable for low-temperature ground storage, while manufactured solid materials (various ceramics) may be widely used for higher temperature storage.
Due to the potential to store thermal energy within a solar thermal power plant, heat storage is currently one of the hot topics to increase the share of solar thermal electricity generation in the future. Furthermore, heat storage application may be highly relevant for small scale heating and cooling in the private sector, large scale heating and cooling in the public and industrial building sector, industrial waste heat storage, as well as heat storage for decentralised CHP systems.
Electrical energy storage
Electrical energy storage stores electrons. In a capacitor, the electricity is stored in the electrostatic field between two electrodes. In superconducting magnetic energy storage (SMES) the electricity is stored in the magnetic field of a coil. The energy capacity is limited, but the reaction time is fast, while the power and efficiency are very high. These methods are still in research and development phase.
POLICY BACKGROUND
- EU climate plan for 2030 and its revision
- Paris Agreement (2015)
- Energy Union Strategy (2015)
- “Clean Energy for All Europeans” package (2016)
- European Parliament (2019): DRAFT REPORT on a comprehensive European approach to energy storage (2019/2189(INI))
- European Green Deal
- European Climate Law COM(2020) 80 final
LIBRARY
- EC (2016): METIS Studies Study S07. The role and need of flexibility in 2030: focus on energy storage
- EASE-EERA (2017): European Energy Storage Technology Development Roadmap 2017
- EC (2017): Follow-up study to the LNG and storage strategy
- EASE (2018): EASE Study on Energy Storage Demand
- Hungarian Gas Storage Ltd (2019): Sustainable Energy Storage Innovations in Danube Region Countries for the EU-Goals of the Paris Climate Agreement
- EC (2020): Study on energy storage – Contribution to the security of the electricity supply in Europe
- EASE (2020): Energy Storage Applications
- Database of the European energy storage technologies and facilities
USEFUL LINKS